Cell Cycle: Do you know that every organism, even the biggest ones, originates from a single cell? How a single cell then develops into such massive entities may be a mystery to you. Cells, and by extension all living things, possess the qualities of growth and reproduction. Every cell divides into two in order to reproduce, producing two daughter cells for every parental cell. A single parental cell and its progeny can expand and divide to establish a new cell population, which is created by these newly born daughter cells growing and dividing. Stated differently, these cycles of growth and division enable a single cell to give rise to a structure made up of millions of cells.
Cell Cycle
In all living things, cell division is a crucial activity. DNA replication and cell expansion also occur during a cell’s division. Therefore, for proper cell division and the creation of offspring cells with complete genomes, all three of these processe, cell growth, DNA replication, and division must occur in unison. The word “cell cycle” refers to the series of processes that occur when a cell duplicates its genome, synthesizes its other components, and ultimately splits into two daughter cells. While cytoplasmic expansion in cells is a constant process, DNA synthesis only takes place at a single phase of the cell cycle. During cell division, a complicated chain of events distributes the duplicated chromosomes (DNA) to daughter nuclei. These occurrences are controlled by genetics.
Phases of Cell Cycle
Human cells in culture depict a normal eukaryotic cell cycle. These cells divide once every twenty-four hours or so. However, the length of the cell cycle can differ between different types of cells and between different organisms. For instance, yeast may complete one round of the cell cycle in roughly ninety minutes.
There are two fundamental phases to the cell cycle:
Interphase
M Phase (Mitosis phase)
The interphase is the period of time between two successive M phases, and the M Phase is the phase during which real cell division or mitosis takes place. It is important to remember that cell division proper only lasts for around an hour during the 24-hour cell cycle of a human cell. For about 95% of the cell cycle, the interphase lasts.
The M Phase begins with nuclear division, which is equivalent to daughter chromosome separation (karyokinesis), and typically concludes with cytoplasmic division (cytokinesis). Despite being referred to as the resting phase, the interphase is when the cell grows and replicates its DNA in an orderly fashion in order to get ready to divide. Three further stages are separated from the interphase:
Phase G1 (Gap 1)
Phase S of Synthesis
Phase G2 (Gap 2)
The G1 phase is the time between the start of DNA replication and mitosis. The cell develops continually and is metabolically active during the G1 phase, but it does not duplicate its DNA. The synthesis phase, or S phase, denotes the time frame in which DNA replication or synthesis occurs.
The amount of DNA in each cell doubles throughout this period. DNA has a starting concentration of 2C, which grows to 4C. There is, however, no increase in the number of chromosomes; if the cell has diploid, or 2n, number of chromosomes at G1, the number of chromosomes stays the same, i.e., 2n, even beyond S phase.
In animal cells, centrioles duplicate in the cytoplasm while DNA replication starts in the nucleus during the S phase. While cell development proceeds, proteins are synthesized in the G2 phase in preparation for mitosis.
Adult animals have numerous cells that only sporadically proliferate, such as heart cells, and many other cells that only divide when necessary to replace lost cells due to injury or cell death. These cells that stop dividing at this point in the cell cycle, known as the quiescent stage (G0), leave the G1 phase. At this stage, cells continue to function as organelles, but they stop proliferating unless the organism requires them to.
Only the diploid somatic cells in mammals exhibit mitotic cell division. There are a few instances, such as male honey bees, when haploid cells divide by mitosis, but these are the exceptions. In response, both haploid and diploid cells in the plants are capable of displaying mitotic divisions. Determine the types of plants and stages at which haploid cells undergo mitosis based on your memory of instances of plant generations alternating. ‘Cell Cycle and Cell Division’
M-Phase (Mitosis Phase)
This stage of the cell cycle is the most dramatic because it involves a significant rearrangement of almost every cell component. It is also known as equational division since the offspring and parent cells have the same amount of chromosomes. Although mitosis has been conveniently split into four nuclear division stages (karyokinesis), it is crucial to realize that cell division is a progressive process and that distinctions between different stages cannot be made with great precision. Four steps are involved in kyokinesis:
Prophase
Metaphase
Anaphase
Telophase
Prophase: The prophase, the initial phase of mitosis’ karyokinesis, comes after the interphase’s S and G2 phases. The newly synthesized DNA molecules are entangled rather than separate during the S and G2 stages. The beginning of chromosomal material condensation indicates prophase. Chromatin condensation is the process that untangles the chromosomal material (Figure). After undergoing duplication during the S phase of interphase, the centrosome now starts to migrate in the direction of the cell’s poles. It is therefore possible to identify the prophase’s conclusion by the following distinctive events:
- Compact mitotic chromosomes are created when chromosomal material condenses. Two chromatids joined at the centromere to form a chromosome are observed.
- The centrosome, which underwent duplication during interphase, starts to migrate in the direction of the cell’s poles. Asters, which are microtubules, are released by every centrosome. The two asters constitute the mitotic apparatus along with the spindle fibers.
Under a microscope, prophase-ending cells lack the nuclear membrane, golgi complexes, endoplasmic reticulum, and nucleolus.
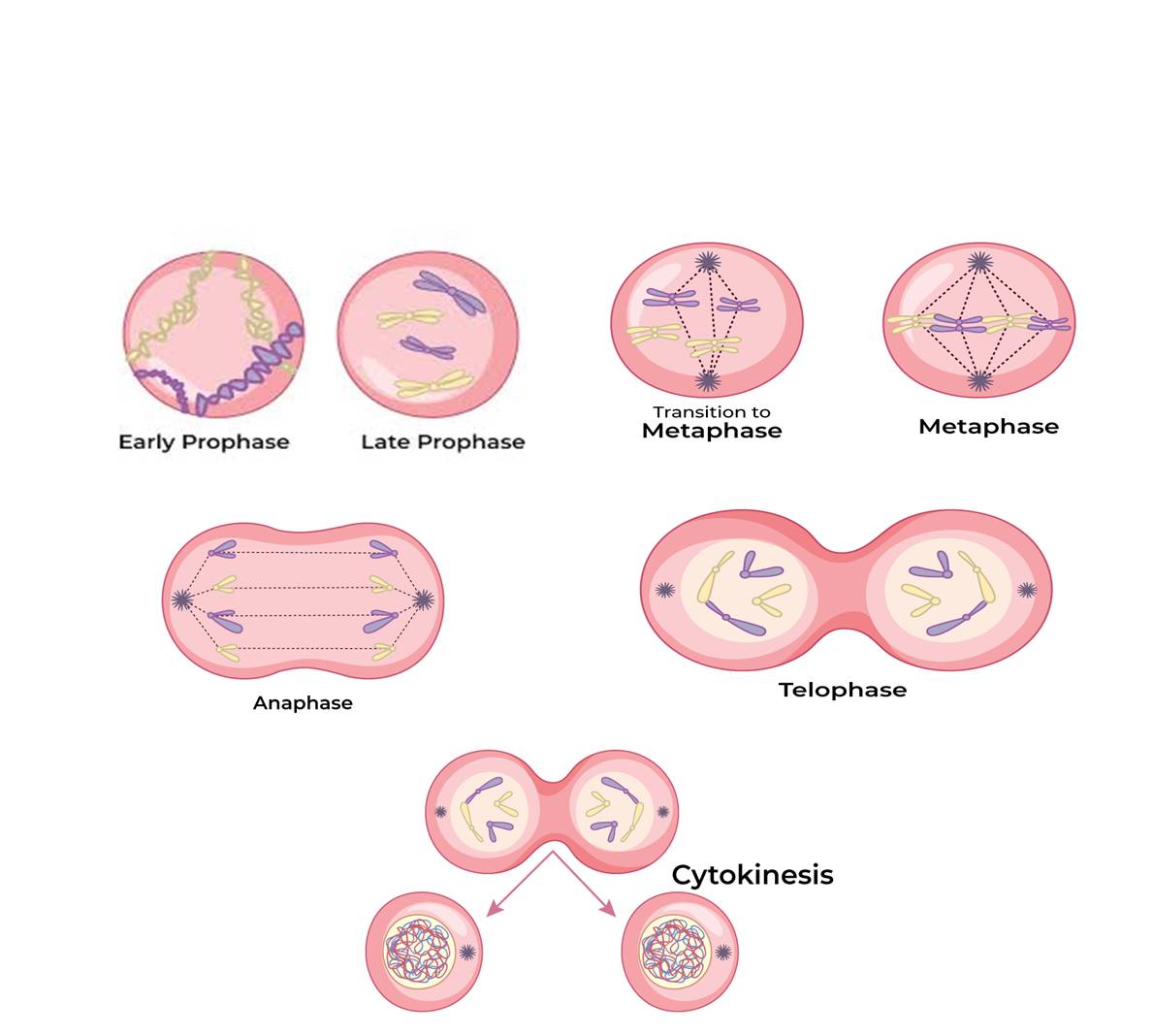
Metaphase: The chromosomes are dispersed throughout the cell’s cytoplasm when the nuclear envelope completely disintegrates, signaling the beginning of the second phase of mitosis.
At this point, chromosomal condensation is complete, allowing for clear microscopic observation. Therefore, this is the stage at which studying chromosomal morphology is easiest. The centromere holds the two sister chromatids that make up the metaphase chromosome at this point together (Figure). Kinetochores are tiny, disc-shaped structures found on the centromere surface. These structures act as the points of connection between the chromosomes that are shifted into place at the center of the cell and the spindle fibers that form those fibers. Since each chromosome has one chromatid connected by its kinetochore to spindle fibers from one pole and its sister chromatid connected by its kinetochore to spindle fibers from the opposite pole, the metaphase is characterized by all the chromosomes coming to lay at the equator (Figure). The term “metaphase plate” refers to the chromosomal alignment plane during metaphase. Key characteristics of metaphase include:
Spindle fibers cling to chromosomal kinetochores.
After being transported to the spindle equator, chromosomes orient themselves along the metaphase plate to both poles via spindle fibers.
Anaphase: Each chromosome present at the metaphase plate splits simultaneously at the start of anaphase, and the two daughter chromatids—which are now known as the daughter chromosomes of the future daughter nuclei—move in opposite directions to the poles. The centromere of each chromosome stays pointed toward the pole and is therefore at the leading edge as it advances away from the equatorial plate, while the arms of the chromosome follow behind (Figure). Anaphase is thus distinguished by the following major occurrences like-Centromeres split and chromatids separate and Chromatids move to opposite poles.
Telophase; The chromosomes that have reached their respective poles decondense and become less unique at the start of telophase, the last step of karyokinesis. Each pair of chromatin material tends to accumulate at each of the two poles, and the individual chromosomes are no longer visible (Figure). This stage displays the following significant occurrences:
- When chromosomes group together at opposing spindle poles, they cease to exist as separate entities.
- Two daughter nuclei are formed as the nuclear envelope grows around the chromosome clusters at each pole.
- Nucleolus, golgi complex and ER reform.
Cytokinensis: In addition to separating duplicate chromosomes into daughter nuclei, or karyokinesis, mitosis also divides the cell into two daughter cells by separating the cytoplasm, or cytokinesis, at the end of which cell division is completed (Figure). In animal cells, this is accomplished by the appearance of a furrow in the plasma membrane, which gradually deepens and eventually joins in the center, dividing the cell cytoplasm into two. Since plant cells are enclosed by a relatively inextensible cell wall, however, they undergo cytokinesis through a different mechanism; in plant cells, wall formation begins in the center of the cell and grows outward to meet the preexisting lateral walls.
Significance of Mitosis
Mitosis is the process by which a cell divides into two identical daughter cells. It is essential for the growth and development of multicellular organisms, as well as for the repair of damaged tissues.
Growth and development: Mitosis allows multicellular organisms to grow and develop by increasing the number of cells in the body. This is important for both embryonic development and postnatal growth.
Tissue repair: Mitosis is also essential for the repair of damaged tissues. When a tissue is damaged, the cells in the surrounding area begin to divide rapidly to replace the lost cells. This helps to heal wounds and prevent infection.
Asexual reproduction: In some organisms, mitosis is the only type of cell division that occurs. This is known as asexual reproduction. Asexual reproduction allows organisms to produce offspring quickly and easily, without the need for a mate.
Meiosis:
Two gametes, each with a full haploid set of chromosomes, fuse together to produce offspring through sexual reproduction. Specialized diploid cells give rise to gametes. This type of specialized cell division produces haploid daughter cells by halving the number of chromosomes. We refer to this type of division as meiosis. While fertilization restores the diploid phase, meiosis guarantees the formation of the haploid phase in the life cycle of sexually reproducing organisms. The key features of meiosis are as follows:
Meiosis is characterized by a single cycle of DNA replication but two consecutive cycles of nuclear and cell division known as meiosis I and meiosis II.
After the parental chromosomes have replicated to form identical sister chromatids at the S phase, meiosis I has started.
Homologous chromosomal pairing and recombination between non-sister homologous chromatids are two processes that occur during meiosis.
Meiosis II culminates in the formation of four haploid cells.
The following steps can be used to categorize meiotic events: Meosis I and Meiosis II
Meosis I:
Prophase I: In comparison to the prophase of mitosis, the prophase of the first meiotic division is usually lengthier and more intricate. Based on chromosomal behavior, it has been further split into the following five phases: diplotene, leptotene, zygotene, pachytene, and diakinesis. Under a light microscope, the chromosomes gradually become visible during the leptotene stage. Chromosome compaction lasts the entire leptotene. The prophase I second stage, known as zygotene, comes next. Chromosomes begin to pair during this stage; this association process is known as synapsis. Homologous chromosomes are a type of paired chromosome. At this point, electron micrographs show that the creation of a complex structure known as the synaptonemal complex occurs concurrently with chromosome synapsis. Tetrads, or bivalents, are complexes made up of two synapsed homologous chromosomes. At the following level, though, they become more apparent. In comparison to the pachytene stage that follows, the first two phases of prophase I are quite brief. At this point, each bivalent chromosome’s four chromatids separate and become evident as tetrads. Recombination nodules, which are locations where non-sister chromatids of homologous chromosomes cross across, become visible during this stage. Genetic material is exchanged during crossing over between two homologous chromosomes. Recombinase is the enzyme that facilitates crossing over, which is another process that is mediated by an enzyme. Genetic information on the two chromosomes recombines as a result of crossing over. As pachytene comes to a conclusion, homologous chromosomes recombine, leaving the chromosomes connected at the locations of crossing over.
Diplotene is identified by the disintegration of the synaptonemal complex and the tendency of the bivalents’ recombined homologous chromosomes to split apart, with the exception of crossover sites. Chiasmata are the names for these X-shaped formations. Diplotene can persist for months or years in the oocytes of certain vertebrates.
Diakinesis is the last phase of meiotic prophase I. Chiasmata’s terminalization serves as a marker for this. In order to ready the homologous chromosomes for separation, the meiotic spindle is constructed and the chromosomes are fully condensed during this phase. The nuclear envelope disintegrates and the nucleolus vanishes by the conclusion of diakinesis. The change to metaphase is symbolized by dialakinesis.
Metaphase I: On the equatorial plate, the bivalent chromosomes align. The homologous chromosomes’ kinetochore is where the microtubules from the opposing poles of the spindle attach.
Anaphase I: Sister chromatids stay together at the centromeres, while homologous chromosomes split apart.
Telophase I: This stage is characterized by the reemergence of the nucleolus and nuclear membrane, cytokinesis, and the so-called dyad of cells. The chromosomes do not attain the greatly stretched state of the interphase nucleus, despite the fact that they do frequently undergo some dispersion. Interkinesis is the term for the brief period that occurs between the two meiotic divisions.
DNA replication does not occur during interkinesis. Prophase II, which is considerably simpler than prophase I, occurs after interkinesis.
Meiosis II:
Prophase II: Meiosis II begins right after cytokinesis, typically before the chromosomes reach their maximum length. Meiosis II is similar to a typical mitosis, in contrast to meiosis I. By the end of prophase II, the nuclear membrane is gone. The chromosomes condense once more.
In metaphase II, sister chromatids’ kinetochores are joined to microtubules from opposing poles of the spindle, and the chromosomes align at the equator.
Anaphase II: The sister chromatids are able to migrate toward the opposing poles of the cell through the shortening of microtubules connected to kinetochores, which starts with the simultaneous splitting of each chromosome’s centromere (which was holding them together).
Telophase II: Telophase II is the final stage of meiosis, during which the two sets of chromosomes are once more encased in a nuclear membrane. Cytokinesis then proceeds, producing a tetrad of cells, or four haploid daughter cells.
Significance of Meiosis
Meiosis is the process that allows sexually reproducing organisms to maintain a particular number of chromosomes between generations, despite the act itself paradoxically reducing chromosome number by half. Additionally, it makes the population of organisms more genetically variable from one generation to the next. Variations play a critical role in the evolutionary process.